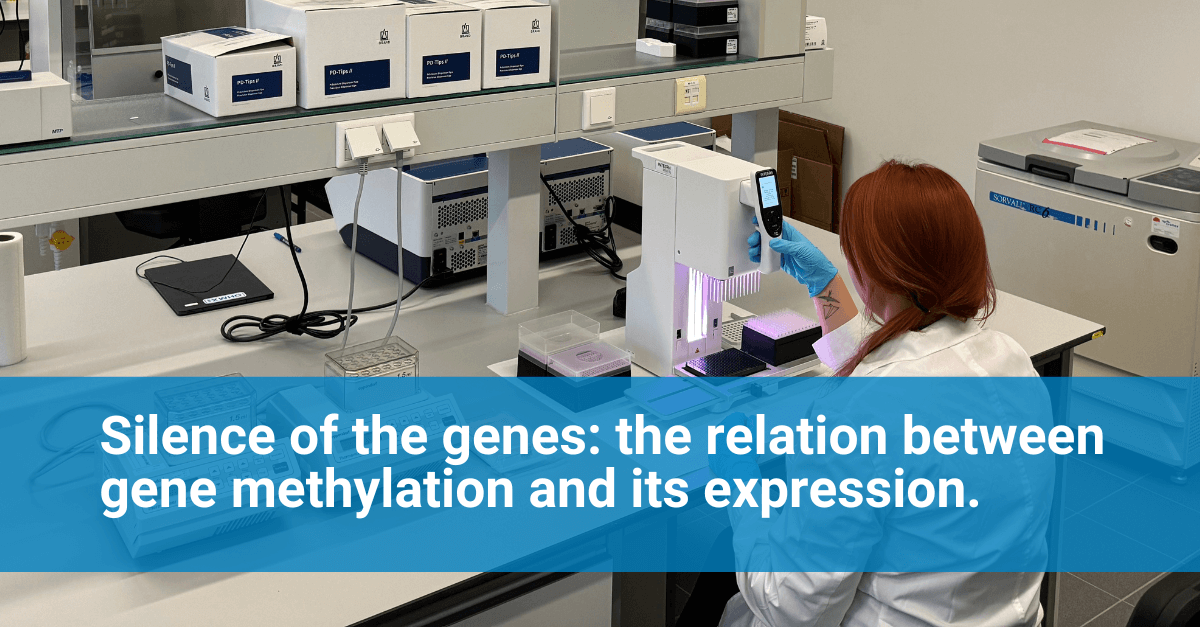
03 Apr Silence of the genes: the relation between gene methylation and its expression.
Methylation as an epigenetic mechanism
At its core, DNA methylation is an epigenetic mechanism that involves the addition of methyl groups to cytosine residues in DNA, thereby affecting gene expression without altering the nucleotide sequence itself (see Figure 1). The methyl group creates a barrier, over which the transcription mechanisms, such as RNA polymerase, have difficulties progressing. This is especially true when promotor regions of genes are methylated. Thus, more methylation (hypermethylation) essentially silences the gene, while no methylation (hypomethylation) would allow the gene to be transcribed and expressed.
Methylation plays a big role in the gene regulation during e.g. embryonic development, regulating the moment when which gene needs to be activated. However, in most fully grown organisms, methylation still plays a dynamic role where genes can be methylated or demethylated depending on the cellular needs, maintaining cellular function and identity. Additionally, the role of non-coding RNAs in epigenetic regulation highlights the complexity of gene expression control. These molecules, which do not code for proteins, can influence gene activity through mechanisms such as RNA interference and the modulation of chromatin structure.
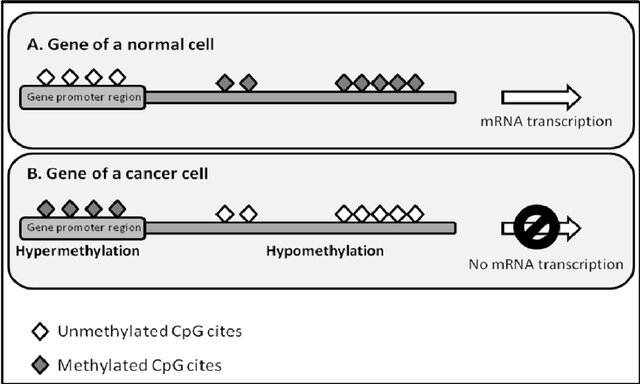
Figure 1: Different methylation patterns in normal (A) and malignant (B) cells. Cancer cells can exhibit global hypomethylation of the genome accompanied by region-specific hypermethylation. Figure copied from Wentzel & Pretorius, 2012 [1]
Faulty methylation patterns
Aberrant methylation patterns are a hallmark of many cancers through inappropriate silencing of tumor suppressors or expression of oncogenes, thus contributing to the development of disease states [2]. A recent multi-omic study [3] investigating methylation patterns of 687 tumor profiles found several clear hyper- and hypo-methylated regions that can be linked to specific cancer types. For instance, prostate cancer can be linked to novel epigenomic subtypes associated with hypermethylation and somatic mutations in TET2, DNMT3B, IDH1, and BRAF [4]. Interestingly, this was discovered using whole-genome, whole-methylome, and whole-transcriptome sequencing. This makes sense, if one wants to truly understand cancer, one must look for different expression patterns and its genomic of epigenomic causes. Understanding these mechanisms could open new avenues for therapeutic interventions, where modulating DNA methylation patterns could restore normal gene function.
Investigating DNA methylation
Methylation patterns in genomes are discovered through a spectrum of techniques ranging from gene-specific approaches to whole-genome analyses. Initially, methods like bisulfite sequencing have been pivotal, offering precise detection of methylated cytosines in individual genes. Moving towards a broader scope, technologies like Reduced Representation Bisulfite Sequencing (RRBS) focussed on CpG-rich regions, providing insights into methylation patterns across specific genomic loci. Advancements in microarray-based methods, such as Methylated DNA Immunoprecipitation Sequencing (MeDIP-seq) and Methyl-CpG binding domain sequencing (MBD-seq), enabled genome-wide methylome profiling, capturing methylated DNA fragments for analysis. Additionally, whole-genome bisulfite sequencing (WGBS) offered comprehensive coverage of methylation patterns across the entire genome.
Long-read sequencing utilizing nanopore technology has emerged as a breakthrough, sequencing genomic and epigenomic (bimodal) data at single-molecule resolution at the same time. This revolutionary approach facilitates discernment of genomic and epigenomic underpinnings of diseases like cancer, offering unprecedented insights into their etiology and progression. Combined with a transcriptome analysis using long-read sequencing, one can combine the (epi)genomic data with expression. Notably, even RNA methylation can be investigated using long-read sequencing [5], adding an additional layer of information to the investigation.
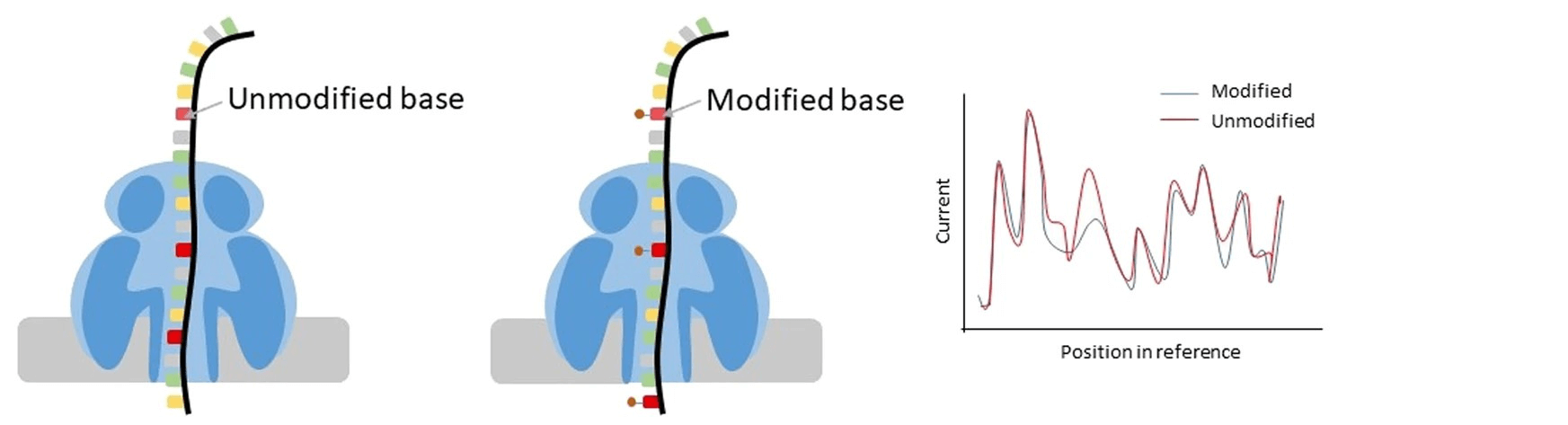
Figure 2: Using Nanopore sequencing to detect modified bases (DNA or RNA) using a difference in current. Machine Learning algorithms are trained to interpret differences in currents while at the same time keeping information on genomic data. These algorithms can be trained for several, if not all, possible base modifications. Figure adapted from Xu & Seki 2020 [6]
The OHMX.bio approach
OHMX.bio has a specialty lab focused on several sequencing solutions. This includes both short- and long-read sequencing with a goal to provide a one-stop solution for your research. OHMX.bio can combine genomic and epigenomic data, sequenced in-house, with transcriptomic and even translatomic and proteomic data. This means OHMX.bio can provide complete information about the pathway of a gene, from its exact genetic code (and mutations) to its regulation, expression, and translation.
The advantage of using one lab to perform all analyses, in a pick-and-mix fashion, is the guarantee that all samples are processed within the same quality system, by the same experts. This leads to fewer logistic errors and paperwork and allows for clear answers. Whether it is for oncological research or biomarker discovery, OHMX.bio can help.
Do you have questions about gene methylation and its expression?
Fill out the form below and our experts will get back to you as soon as possible!
References
[1] Wentzel, Jaco & Pretorius, Piet. (2012). Investigating the Role DNA Methylations Plays in Developing Hepatocellular Carcinoma Associated with Tyrosinemia Type 1 Using the Comet Assay. 10.5772/2159.
[2] Lakshminarasimhan R, Liang G. The Role of DNA Methylation in Cancer. Adv Exp Med Biol. 2016;945:151-172. doi: 10.1007/978-3-319-43624-1_7. PMID: 27826838; PMCID: PMC7409375.
[3] Wen-Wei Liang et. al. Integrative multi-omic cancer profiling reveals DNA methylation patterns associated with therapeutic vulnerability and cell-of-origin, Cancer Cell, 41, 9, 2023:1567-1585.e7, ISSN 1535-6108, https://doi.org/10.1016/j.ccell.2023.07.013.
[4] Zhao, S.G., Chen, W.S., Li, H. et al. The DNA methylation landscape of advanced prostate cancer. Nat Genet 52, 778–789 (2020). https://doi.org/10.1038/s41588-020-0648-8
[5] An, Y., Duan, H. The role of m6A RNA methylation in cancer metabolism. Mol Cancer 21, 14 (2022). https://doi.org/10.1186/s12943-022-01500-4
[6] Xu, L., Seki, M. Recent advances in the detection of base modifications using the Nanopore sequencer. J Hum Genet 65, 25–33 (2020). https://doi.org/10.1038/s10038-019-0679-0